Thermophotovoltaics
May 30, 2022
I once
researched a
thermoelectric device for
energy recovery of the
waste heat of
aircraft turbine engines. Prior to that, I had never been interested in thermoelectric devices because of their low
efficiency. I was also
annoyed that even modest increases in their efficiency were
praised in
press releases for no reason other than as a way of generating more
research funding. Thermoelectrics were only useful in my particular application because of the large
temperature gradient involved. Turbine engines run really hot, and the temperature difference to a
coolant, such as
air, is huge. I wrote about thermoelectrics in an
earlier article (Thermoelectrics, October 26, 2020).
A simple
thermoelectric generator, as shown in the figure, operates by the
Seebeck effect, named after
Thomas Seebeck (1770-1831). Seebeck demonstrated that dissimilar
conductors will generate
electricity when a temperature gradient is applied. The
principle is that the
heat flow in a conductor caused by a temperature gradient results in a diffusion of
charge carriers, and this becomes a
voltage difference.
Thermocouples, useful to
temperature measurement, are devices that use
metal pairs of
alloys such as
chromel-
alumel (
Type K thermocouple). Shown in the figure is a Seekbeck device that operates by
electron and
hole transport in
semiconductors.
A thermoelectric cell of n- and p-doped semiconductor.
A series connection of many such cells offer a means to extract useful electrical power from sources of waste heat, such as automobile exhaust gas.
(Click for larger image.)
Since electrons contribute to both
thermal conductivity and
electrical conductivity, it's difficult maintaining a large thermal gradient in thermoelectric materials. However,
certain semiconductors have a high
ratio of electrical conductivity to thermal conductivity.
Junctions of
n- and
p-doped bismuth telluride (Bi2Te3) are typically used in thermoelectric cells, and there are
nanotechnology techniques to further increase thermoelectric efficiency.
Good thermoelectric
performance is identified by a high
Seebeck coefficient (
S,
volts/
kelvin), and low
thermal conductivity (κ,
watts/(
meter-kelvin)); so, these are combined into a
thermoelectric figure of merit, commonly denoted as
zT, as follows:
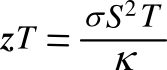
In this
equation,
σ is the
electrical conductivity. Somewhat confusingly,
zT, is used as the figure of merit, since
multiplication by
temperature gives a
dimensionless number. That's why we don't just cancel the temperature term from both sides of the equation. The highest figures of merit were stalled at just slightly above 1.0 with no great advances over the years. However, the figure of merit has approach 2.0 in
decades of research, and it's now possible to get an energy conversion efficiency of nearly 10%.
Research continues on thermoelectric materials, since there are so many applications for thermoelectric recovery of waste heat in today's
energy-conscious world. As I write this, my
desktop computer is blowing heated air into my
office, which is a benefit on
cold winter nights, but a thermoelectric generator could reduce my
carbon footprint on
hot summer days.
Thermoelectric efficiency is still too low, so it was refreshing to read that a research team from
MIT and the
National Renewable Energy Laboratory (NREL) has enhanced the efficiency of a different device for conversion of heat to electricity. Their
thermophotovoltaic (TPV) cell captures
high-energy photons from a
white-hot heat source and
converts that
light to electricity, just as a
photovoltaic solar cell converts
sunlight into electricity.[1-3] This device operates best with a very high temperature source, around 2000
°C. It would have been more useful in my turbine engine application than a thermoelectric generator, but it won't be useful for general waste heat recovery from the most common sources. It's intended as a means of
storing energy by heating a
material such as
graphite to recover the energy at a later time.[1-3]
The MIT thermophotovoltaic cell.
This 1 cm x 1 cm cell is mounted on a heatsink for an efficiency measurement.
(MIT image by Felice Frankel, licensed under a Creative Commons Attribution Non-Commercial No Derivatives license.[2] Click for larger image.)
The device, which generates electrical power from a heat source of between 2,900 to 2,400
degrees Celsius, has more than a 40% efficiency.[2] This efficiency is about that of
steam turbine generator, which is in the range 33-48%.[2]
Thermodynamics places an upper efficiency limit on steam conversion at 60%.[2] Presently, more than 90 percent of
global electricity derives from heat sources such as
coal,
natural gas,
nuclear energy, and
concentrated solar energy, with the conversion of heat to electricity via steam turbine generators.[2] This device, which operates as a heat converter with no moving parts, can work at higher temperatures and at a potentially higher efficiency. Says the
paper's corresponding author,
Asegun Henry of the
MIT Department of Mechanical Engineering,
"One of the advantages of solid-state energy converters are that they can operate at higher temperatures with lower maintenance costs because they have no moving parts... They just sit there and reliably generate electricity."[2]
Thermophotovoltaic devices with just a few percent efficiency were first fabricated in the
1960s, but the efficiency increased to a record 32% in 1980 and plateaued.[2-3] The reason for lack of progress is that heat
radiation, idealized as
blackbody radiation, extends over a wide range of
wavelengths, while photovoltaics are optimized for photon conversion in a narrow range of wavelengths, and some of the light energy is wasted.[3] This new thermophotovoltaic device captures more of the light
spectrum through use of
wide-bandgap semiconductors stacked in
layers that allow photon
absorption over an extended range of wavelengths.[2]
blackbody radiation curves.
Higher temperatures are characterized by greater energy and a shift to lower wavelengths.
(Modified Wikimedia Commons image by Darth Kule. Click for larger image.)
To achieve such high efficiency, the thermophotovoltaic device was built from more than twenty layers of materials such as
GaAs,
(Al,Ga)As,
(Ga,In)P,
(Ga,In)As,
(Al,Ga,In)As, and
Ga(As,Sb).[1] This created two separate photovoltaic cells, stacked one on top of the other, in which the top cell is an absorber of mostly
visible and
ultraviolet light, while the lower cell handles the
infrared.[2-3] There's a
gold layer at the bottom that
reflects the low-energy photons not absorbed by these two cells, so the energy can be reabsorbed by the heat source.[2-3]
Device testing was accomplished by exposure to concentrated light from a high temperature
lamp.[2] By changing the voltage, they changed the lamp's temperature; and the thermophotovoltaic device maintained an efficiency of near 40 percent from 2,400-2,900 degrees Celsius with a maximum efficiency of 41.1% for a 2400 °C
tungsten filament.[2-3] The thermophotovoltaic cell in this study had an area of a
square centimeter.[2] A
grid-scale thermal battery system would require 20,000
square feet (about a quarter the
area of a
football field).[2]
It's estimated that even a 35% efficiency in heat-to-electricity conversion would make this thermal battery approach to energy storage
economically viable.[3] Such a system would use
renewable energy to heat a
liquid metal
bath, or graphite, to the required high temperature.[2-3] A
mirror capable of reflecting nearly 99% of unabsorbed infrared light back into the heat source was developed previously, and this would boost the device efficiency to a 50% level.[3-4] A
venture has been launched to
commercialize this
technology, and it's estimated that the
capital requirement for a viable system would be about $10 per
kilowatt-hour of capacity, less than a tenth the cost of grid-scale
lithium ion batteries.[3] This research was partially
supported by the
United States Department of Energy.[2]
References:
- Alina LaPotin, Kevin L. Schulte, Myles A. Steiner, Kyle Buznitsky, Colin C. Kelsall, Daniel J. Friedman, Eric J. Tervo, Ryan M. France, Michelle R. Young, Andrew Rohskopf, Shomik Verma, Evelyn N. Wang & Asegun Henry, "Thermophotovoltaic efficiency of 40%," Nature, vol. 604 (April 13, 2022), pp.287-291, https://doi.org/10.1038/s41586-022-04473-y. This is an open access article with a PDF file here.
- Jennifer Chu, "A new heat engine with no moving parts is as efficient as a steam turbine," MIT Press Release, April 13, 2022.
- Robert F. Service, "'Thermal batteries' could efficiently store wind and solar power in a renewable grid," Science, vol. 376, no. 6590 (April 15, 2022), p. 230.
- Dejiu Fan, Tobias Burger, Sean McSherry, Byungjun Lee, Andrej Lenert, & Stephen R. Forrest, "Near-perfect photon utilization in an air-bridge thermophotovoltaic cell," Nature, vol, 586 (September 21, 2020), pp.237-241, https://doi.org/10.1038/s41586-020-2717-7.
Linked Keywords: Research; researched; thermoelectric effect; electronic component; device; energy recovery; waste heat; aircraft; gas turbine; turbine engine; energy conversion efficiency; annoyance; annoy; praise; praised; press release; funding of science; research funding; temperature gradient; coolant; atmosphere of Earth; air; thermoelectric generator; Seebeck effect; Thomas Seebeck (1770-1831); electrical conductor; electricity; physical law; principle; heat transfer; heat flow; charge carrier; voltage; thermocouple; temperature measurement; metal; alloy; chromel; alumel; Type K thermocouple; electron; electron hole; charge transport; semiconductors; thermoelectric generator; thermoelectric cell; n-type semiconductor; p-type semiconductor; series circuit; series connection; electric power; automobile; exhaust gas; thermal conductivity; ratio; p-n junction; bismuth telluride (Bi2Te3); nanotechnology techniques; device efficiency; device performance; volt; kelvin; watt; meter; thermoelectric figure of merit; equation; multiplication; temperature; dimensionless number; decade; energy crisis; energy-conscious world; desktop computer; office; cold; winter; night; carbon footprint; hot; summer; day; Massachusetts Institute of Technology; MIT; National Renewable Energy Laboratory (NREL); thermophotovoltaic (TPV); cell; high-energy photon; incandescence; white-hot; heat source; energy transformation; energy conversion; light; photovoltaic; solar cell; sunlight; Celsius; °C; energy storage; storing energy; material; graphite; Felice Frankel; centimeter; cm; heat sink; heatsink; measurement; Creative Commons Attribution Non-Commercial No Derivatives license; degrees Celsius; steam-electric power station; steam turbine generator; thermodynamics; world economy; global; coal; natural gas; nuclear power; nuclear energy; concentrated solar power; concentrated solar energy; scientific journal; paper; corresponding author; Asegun Henry; MIT Department of Mechanical Engineering; solid-state (electronics); maintenance, repair and operations; cost; reliability (engineering); reliably; 1960s; electromagnetic radiation; black-body radiation; blackbody radiation; wavelength; electromagnetic spectrum; wide-bandgap semiconductor; coating; layer; absorption (electromagnetic radiation); Planck's law; curve; Wikimedia Commons; Darth Kule; gallium arsenide; GaAs; aluminium gallium arsenide; (Al,Ga)As; indium phosphide; (Ga,In)P; indium gallium arsenide; (Ga,In)As; aluminium gallium arsenide; (Al,Ga,In)As; gallium antimonide; Ga(As,Sb); visible spectrum; ultraviolet; infrared; gold; reflection (physics); reflects; incandescent light bulb; lamp<; tungsten; filament; square centimeter; electrical grid; grid-scale; square foot; square feet; area; American football field; economics; economically; renewable energy; liquid; thermal reservoir; bath; mirror; venture capital; commercialization; commercialize; technology; capital (economics); kilowatt-hour; lithium ion battery; supported; United States Department of Energy.